Over the last years, research areas such as interplay between tectonics and sedimentation in rift systems, salt tectonics, pre-Jurassic petroleum systems, and the subsurface geology of the high north have contributed to highlight new petroleum exploration play concepts on the Norwegian Continental Shelf (NCS).
Blog post
The blogpost is written by
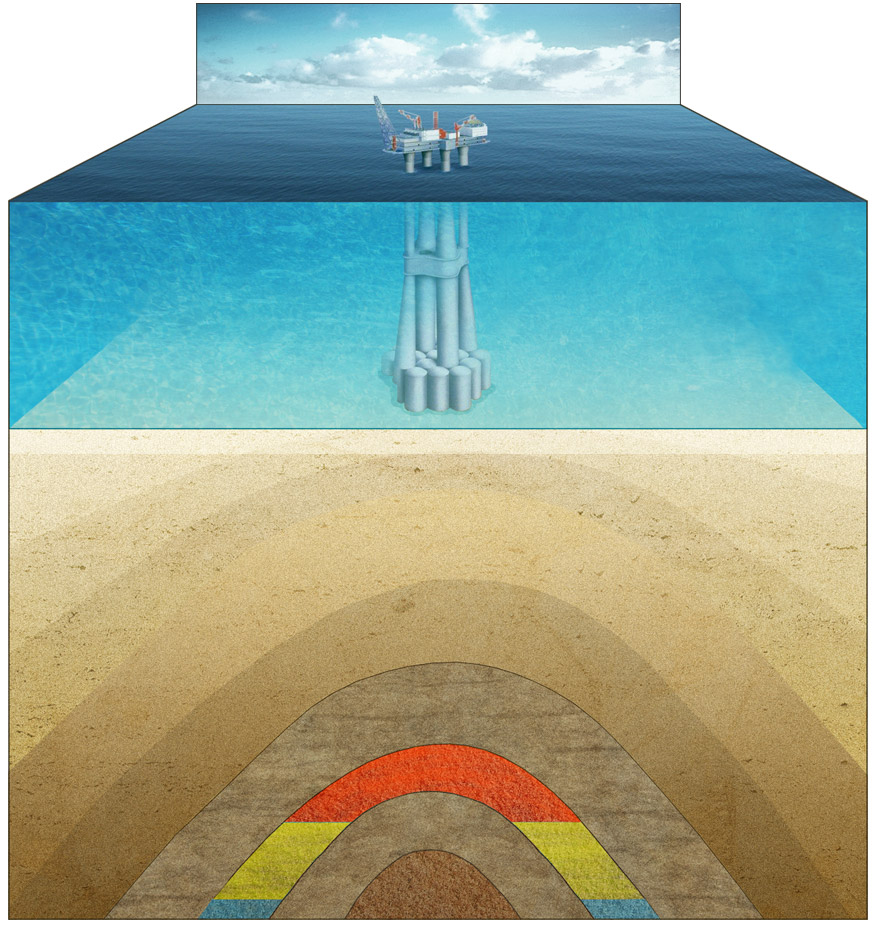
Recent discoveries are small, most of them near existing infrastructure and many plays are still poorly understood. Traditionally, exploration and exploitation of NCS reservoirs have been limited to hydrocarbon production. Therefore, a holistic resource evaluation of the subsurface as a comprehensive energy system, given its large potential for renewable energy supply and storage, will add value, provide energy security, and contribute to the transition. For example, non-traditional reservoirs, such as salt caverns (Caglayan et al., 2020), for hydrogen storage, and high-pressure high-temperature (HPHT) reservoirs for geothermal energy (Cui et al., 2016) are key for the transition and should be further studied.
Digitalisation of the reservoir
Today’s development towards digitalisation, big data and machine learning (ML) can provide new tools to maximise data utilisation and extract more information for improved subsurface understanding and for realistic modelling of matured areas. This knowledge can be utilised not only for hydrocarbon exploration and production, but also for CO2 and H2 storage and geothermal energy extraction.
However, there is a lack of quantitative data-driven understanding of various key risk factors, and proper integration of data for improving reservoir understanding. The current understanding of hydrocarbon charging of the reservoirs is the main uncertainty, reflecting our limited capability in mapping the distribution of source rocks and fluid migration (NPD, 2020). In this context, aquifer behaviour and connectivity play an important role and is critical for storage capacity of energy and waste.
The energy mix scenario
Improved understanding of the migration and distribution of fluids will open undiscovered potential in near-field areas not only for hydrocarbon but also for other fluids and improve well placement. The energy mix scenario is based on clean H2 production combined with large-scale CCS and underground H2 storage. Several pilots and small-scale industrial projects have substantially increased confidence in underground CO2 and H2 storage reliability. However, gigaton scale CO2 injection has not been implemented on the NCS, and many challenges remain, e.g. large injection rates could bring significant geomechanical effects (Elenius et al., 2018; Wangen et al., 2016). CO2 mixed from multiple sources raises a question of CO2 quality and the effect of impurities on the reservoir flow and seal integrity. Existing reservoir simulation tools are struggling to provide reasonable predictions for CO2 flow (Räss et al., 2014; Verdon et al., 2013). Attempts have been made to history-match observations at the Sleipner field using conventional reservoir simulations. However, these have failed to capture the first-order observations, such as facile vertical flow of CO2 through low permeable shale layers, the formation of focused fluid flow channels or chimneys, and rapid lateral spreading underneath the seal rock (Boait et. al., 2012).
Hydrogen storage
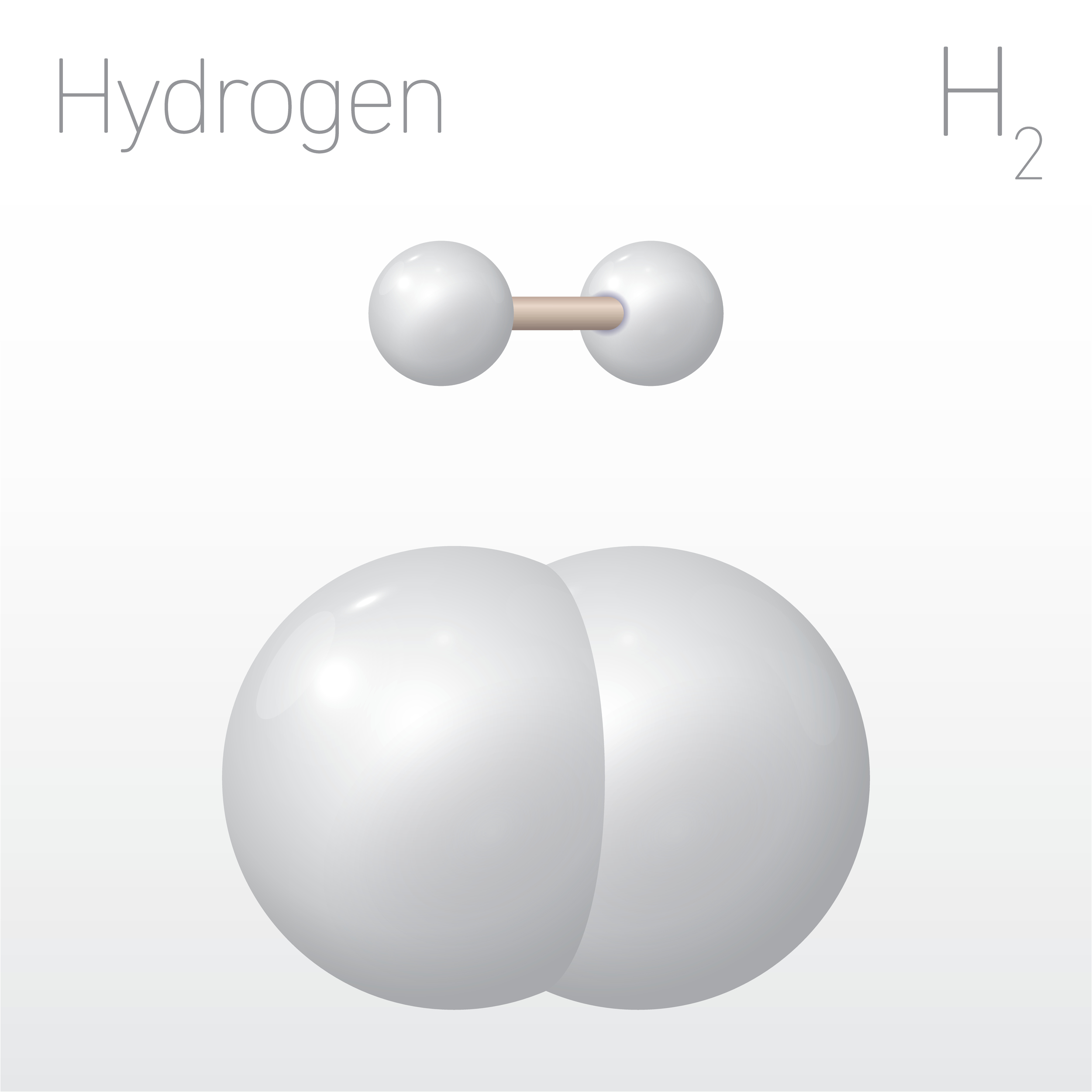
Furthermore, the underground capacity for H2 storage in salt caverns, and porous reservoirs such as saline aquifers or depleted gas reservoirs is still in its infancy. Technical feasibility, efficiency, and safety of H2 storage are impacted by fluid-rock interactions meaning that a coupled hydro-chemo-mechanical approach is required to assess the short- and long-term impact of cyclical H2 injection. The effect of fluid-rock interactions on storage integrity or capacity is currently unknown and needs an interdisciplinary approach of experimental investigations, numerical simulations, geological characterization and assessment of operational procedures. On the other hand, the potential for geothermal energy is large in areas with low-temperature gradients, although there are currently few sites in operation globally (IGA, 2014). Improvements in drilling technologies bring new opportunities for developing geothermal energy in Norway by use of, e.g., closed geothermal systems with a coaxial heat exchanger. However, these technologies are still under development, and further feasibility studies are needed.
EOR methods
Existing fields are on decline and producing at high water-cut, which increases energy demand, costs and emissions per barrel with time. Enhanced oil recovery (EOR) methods to further improve reservoir sweep and to minimize water/gas-cut may have large potential to optimise water and gas injection and reservoir sweep efficiency leading to accelerated production and reduced energy needs and emissions. Recent knowledge acquired from various research groups has established fundamental knowledge of the NCS reservoirs and their improved recovery potentials. Methods such as low salinity (Smart Water), alone or in combination with polymers, hydrocarbon gas or CO2 miscible gas/WAG injection, have been identified with high technical potential and economically feasible (Smalley et al., 2020).
Comprehensive research, largely at the National IOR Centre of Norway and by its partners, forms a solid foundation for the future net-zero emission production. These include:
- effects of pore fluid chemistry and temperature on wetting and geomechanical properties of reservoir rock
- improved oil-water mobility ratio by combining Smart Water and polymer and by wettability alteration
- CO2-WAG or CO2-foam for mobility control combined with CO2 storage
- accelerated and incremental oil recovery from sandstone and carbonate reservoirs with Smart Water
- carbonated water to improve water flooding performance while simultaneously storing CO2
- reservoir monitoring with partitioning tracers
- environmental risk assessment for EOR
- CO2 EOR for tight reservoirs
- modelling tool IORSim for EOR processes
- better prediction of IOR potential
- Open Porous Media (OPM) for reservoir management
EOR as a tertiary recovery method often requires additional equipment and chemicals that are energy- and cost-demanding, leading to added emission. Methods that accelerate production and store CO2 at the same time (when used as injectant, or combined with late field life CO2 storage), will be prioritized by NCS2030. Methodologies for accounting of the reduced green house gas emissions and energy saving in the complete EOR life cycle must be developed (Metidji, 2021). Most of the earlier research has been conducted at the nano- to core-scale and rely on models to predict field scale potential. Recent large-scale testing and field testing for foam-based CO2 injection have demonstrated the need for more large- and pilot-scale testing to upscale the EOR methods for offshore fields.